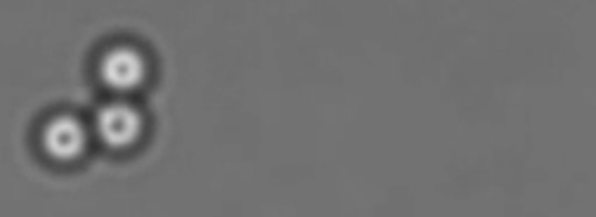
How microscopic scallops wander
All microscopic objects, from enzymes to paint particles, are jittering constantly, bombarded by solvent particles: this is called Brownian motion. How does this motion change when the object is flexible instead of rigid? Ruben Verweij, Pepijn Moerman and colleagues published the first measurements in Physical Review Research.
Botanist Robert Brown gave his name to the jittery motions he saw pollen grains make when he studied them in 1827 under his microscope. Since then, the properties of Brownian motion have been intensively studied: they are caused by fast-moving water molecules bumping into the slower-moving particles, as was explained by Einstein and Perrin in the early 1900s.
Brownian motion also affects biological microscopic particles, such as enzymes, RNA, and antibodies. Most of these are not rigid but flexible: they can change their shape and hence, their function.
How does that affect their Brownian motion? Predictions made in the 1980s could not be tested for a long time, because experimental model systems with well-defined shape changes, large enough to be observed, did not yet exist.
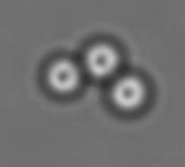
Micrometre-sized beads
This changes with the publication by Verweij and Moerman, a collaboration with Willem Kegel, Jan Groenewold and Alfons van Blaaderen from Utrecht University. 'We have built the simplest model system imaginable for micrometre-sized flexible objects, which you can also study under a light microscope', says Verweij.
Daniela Kraft’s group uses colloids: micrometre-sized beads which move around in water and can be observed using a microscope. The group developed a method to coat colloids in a lipid bilayer with inserted DNA molecules, which can couple selectively to DNA molecules around another colloid particle. This creates a hinge that can freely change its shape because the lipid bilayer around the particles is fluid.
A series of three colloids, coupled in this way, is the model system. 'It's easy to see the flexibility under the microscope, by tracking the angle that the three make', says Verweij. He filmed about 30 of these triplets while they were diffusing, moving, rotating, and closing and opening under the bombardment of surrounding water molecules.
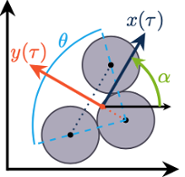
Quasi-scallop mode
The videos were analysed, yielding the first experimental comparison between rigid and flexible Brownian motion. The first result: flexible particles move slightly faster than rigid ones. Verweij: 'It's a small but measurable difference, about three per cent. More importantly, we found certain couplings between shape changes and displacements', says Verweij. The meaning of this is subtle, and Verweij tries to explain. 'When a scallop actively closes its shell, it will move forward in the direction of the hinging point. We found a similar correlation for our tiny hinges, which move only passively, and call it the Brownian quasi-scallop mode.'
Although subtle, the researchers observe a definite statistical correlation between the Brownian opening and closing, and the movement that the triplet makes. These correlations had been predicted, and have now finally been confirmed.
Microhinges diffusing
Due to the selected cookie settings, we cannot show this video here.
Watch the video on the original website orRigid versus Flexible
Finally, the authors studied the effect of time. Flexible trimers in an extended configuration move faster along their long axis than along their short axis, just like rigid particles. For rigid particles, this effect vanishes over time because of their rotational motions. For flexible particles, this process happens faster because they also change shape, causing this preferred direction to even out.
The rate at which this happens, therefore, depends strongly on the flexibility. 'It goes from roughly 30 seconds for rigid particles to 10 seconds for flexible ones', says Verweij.
'Measurements like this are important, since many biological molecules are also flexible, and interactions between them depend on this. For instance, the lock-and-key fitting between a protein and a receptor can be influenced by Brownian shape changes.'

Complex clusters
Moreover, flexible colloid-hinges can be used as models for simple molecules, where atoms are coupled. But while molecules cannot be resolved using a microscope, the colloids can.
The results and the methods may ultimately be useful for research into drugs and diseases, but, stresses Verweij, this is fundamental research, primarily aimed at understanding the underlying physical processes.
'Now we would like to research longer and more complex clusters, for example of four spheres. In that case, there are more degrees of freedom, which of course makes the behaviour even more complex and interesting.
Text: Bruno van Wayenburg